Incorporating Indigenous Technologies into Enhanced Stormwater, Erosion and Sediment Control Systems

The Antamina Mine is located in the mountainous region of the Ancash Department of Peru at an elevation of approximately 4200m. The 120 km main access road crosses the headwaters of numerous high-value trout streams and is adjacent to pristine lakes, some of very high ecological and scenic value. In addition, the mine discharges mine water and tailings supernatant to the Antamina and Ayash watersheds. These streams provide the primary water supply for irrigation by local residents and provide excellent fishery habitat.
The rock walls and earthen diversions constructed by historic and current indigenous residents of Peru are world -renowned and local residents continue this tradition, passed down from the Inca Civilization, for both agricultural purposes and water distribution. The key elements of sustainable development – economic, environmental and social development have been incorporated into Antamina’s sustainable development program through integration of indigenous knowledge and capabilities into enhanced storm water, erosion reduction and sediment control systems.
Examples of incorporating indigenous technologies and knowledge into cost-effective and environmentally sound environmental controls are: (1) placement of rock walls on steep terrain to reduce slope length and erosion, (2) installation of rock walls (porous rock check dams) within elongated sediment ponds to increase detention time, (3) construction of rock-lined ditches to convey clean water (4) use of rock walls, with or without geotextile, to function as a seep berm, and (5) appropriate selection and usage of native Andean species for protection of canals and sediment ponds, and steep slopes.
INDIGENOUS ROCK STRUCTURE TECHNOLOGY
A visit to the Museum of Anthropology in Lima, Peru, illustrates the long tradition of using rock as a mechanism to access fundamental resources. Before ropes and pulleys were invented, a simple structure was in place in Peru, now mimicked in open-pit mining, consisting of a spiraling ramp with hand-placed rock stabilized walls, 1.5 to 2m in height, accessing a dugout water well located 15-plus meters below the ground surface. The well-known tradition of using rock to develop and stabilize trails used for travel and commerce is especially noteworthy along the Inca trail between Cusco and Machu Picchu. Throughout the steep slopes of the Peruvian Andes an agrarian patchwork quilt can be seen, Figure 1, displaying various shades of earth tone green and wheat colors, segmented by rock walls that function as terraces stabilizing farmer’s fields. Rock structures are used to designate property boundaries, provide temporary confinement of animals, form the foundation and lower wall of homes, convey irrigation water and distribute water for domestic supply. Early on in the Antamina project, it became apparent that there was a tremendous opportunity to incorporate this rich culture and knowledge base into the many diverse needs of a modern mining operation. Integration of traditional rock structure techniques with state-of-the-art environmental control technologies results in control systems that are cost-effective and enhance overall performance, and form part of the company’s sustainable development program.
IMPLEMENTING THE PRINCIPLES OF SOCIAL RESPONSIBILITY
Antamina is committed to incorporating social responsibility into all aspects of the project (Botts, 2003). Opportunities to implement the concept of a “triple bottom line” where – economic, environmental and social responsibility are considered key components of business activity are aggressively pursued. Experience has shown that the best strategy for achieving acceptance and support of a large mining project is to actively engage stakeholders in an open and transparent process of consultation, involve communities in decisions and highly integrate the indigenous population thorough incorporating their knowledge and experiences. Such activities: (1) respect the culture, customs and values of individuals and communities, (2) promote conservation of cultural and natural heritage, (3) provide opportunities to hire, and use the skills of, local workers and (4) promotes protection of the environment. Furthermore, opportunities to build safety awareness that can lead to cultural changes over the long term are as such part of the sustainable development program at Antamina. This approach to stakeholder relations is building trust; establishing a real sense of ownership and hence producing project buy-in. Establishing such partnerships and promoting involvement of stakeholders and communities is a goal put into practice at Antamina.
STORMWATER, EROSION REDUCTION AND SEDIMENT CONTROL: OPPORTUNITIES TOINTEGRATE INDIGENOUS TECHNOLOGIES
The Antamina project consists of an open pit mine, a 70,000 ton per day concentrator, a 302 Km. long concentrate pipeline, port facilities, a new access road, power line, and town site. The project is designed to produce up to 1.5 million tonnes per year of copper and zinc concentrates over a 23-year project life, and will be the third largest producer of zinc and the seventh largest producer of copper in the world.
There are many fundamental design concepts that when integrated and implemented into the project’s mitigation strategy, result in enhanced storm water, erosion reduction and sediment control system that is both protective of the environment- and cost-effective (Warner and Torrealba, 2003). Indigenous rock construction techniques were integrated with the state-of-the-art design methodology and technologies to provide a control system at Antamina that has performed with a high degree of reliability and achieved excellent effluent water.
The Sediment, Erosion and Discharge by Computer Aided Design (SEDCAD version 4.0) program was used to design a system of controls for the major watersheds within the Antamina mining complex (Warner, et al., 1998). SEDCAD was used to design and predict the expected performance of both individual controls and the linked system of control technologies with respect to peak flow, runoff volume, erosion rates, and peak inflow and effluent sediment concentrations. Furthermore, SEDCAD enables the prediction of complete hydrographs and sediment graphs, effluent sediment particle size distribution and sediment trap efficiency. Sizing of structures, such as diversion channels, clean water aqueducts, culverts, energy dissipaters, earthen channels, rock-stabilized channels, porous rock check dams, silt fences, porous rock walls, and sediment ponds, with numerous configurations, was accomplished through application of the SEDCAD program.
During the design process, there were many opportunities to incorporate indigenous rock structure technologies used for storm water, erosion reduction and sediment control. In some cases, such as rock-lined channels, integration was straightforward. In many other cases the integration of rock structure techniques were analyzed to enhance the performance of standard control methods.
Besides individual controls, rock wall and rock check dam techniques were used as an integrated and linked systems of controls that functioned to incrementally enhance the overall performance of the system. Furthermore, rock structures enable the linkage of downgradient natural controls, such as stream buffers, to increase treatment efficiency, especially for the very frequent small and medium size storms and during the critical trout spawning timeframe.
ILLUSTRATIVE EXAMPLES OF INCORPORATED ROCK STRUCTURES: DESCRIPTION AND PREDICTED PERFORMANCE
The applicability of integrating rock structures into storm water, erosion reduction and sediment control systems is illustrated through representative examples contained in various watersheds. Along the 120 Km access road, rock diversion channels and rock energy dissipaters were utilized. The Laguna Canrash (Km 94) site required stabilization of a steep (74%) long (240m) slope that was created during road construction. The sediment ponds at Km 97 originally constructed during the construction period were upgraded using porous rock check dams, located within the duel ponds, and also rock structures were used within the weep berm to control the distribution of runoff to a down-gradient natural grass stream buffer strip.
In the Tucush watershed, where a 750,000,000 Mt waste rock dump is planned, rock-lined ditches were employed to convey clean water that would otherwise comingle with sediment-laden runoff, and numerous rock structures were installed to function as a comprehensive system of erosion and sediment controls which included rock walls, rock walls with geotextile, and rock check dams used to provide a series of small ponds. For the active pit area in the Antamina watershed, a clean water aqueduct was designed and constructed and a series of 12 chambers, used to enhance the performance of sediment basin 5, were established through the use of porous rock structures. Predicted performance of various structures is included for a 2-year 24-hour design storm based on the SEDCAD analysis.
EARTHEN AND ROCK DIVERSION CHANNELS ALONG THE 120 KM ACCESS ROAD
Three basic design principles for controlling erosion and sedimentation are: (1) to keep clean water clean, (2) to eliminate runoff from entering and eroded steep slopes and (3) to stabilize all sub-areas at each phase of construction. These basic principles were applied along the entire 120 Km access road. Earthen and rock stabilized diversion channels, primarily using indigenous workers and techniques, were established up-gradient of the cut slope, runoff transferred to concrete-lined or rock down-drains prior to entering a culvert and then an energy dissipater established thorough integrating design procedures with rock placement skills of workers. The use of these rock diversion channels enabled the establishment of cut slope vegetation without the need to continually re-grade slopes to remove gullies. Approximately 2,300 m of rock-lined clean water diversions was established along the120 Km access road. This was accomplished through the employment of 180 local workers at an estimated cost of $ 300,000 (US). Safety training was provided to these local workers and emphasized throughout the construction phase, resulting in 0 loss worker days due to accidents.
STABILIZATION OF A LONG, STEEP FILL SLOPE (KM 94)
For long and steep fill slopes, rock walls were built using traditional Peruvian terrace construction techniques. For this particular situation the primary sediment source area is the steep (74%), long (240m) slope between the main access road and the channel leading to a series of four down-gradient sediment ponds. Spacing between rock walls was based on design analysis that considered tradeoffs between expected performance, with respect to erosion rates, and costs associated with re-vegetation and sediment clean-out requirements of a series of previously established down-gradient ponds that have no access by heavy equipment. Performance considerations included erosion and sedimentation rates, peak flow reduction and effluent sediment concentration.
Antamina conducted an aggressive and extensive slope stabilization and vegetation program covering approximately 8 ha. The slope was initially stabilized through the use of over 2,200m of rock walls that significantly reduced slope length thus reducing erosion rates and also reduced the development of concentrated flow thereby further reducing sediment generation. The runoff temporarily stored up-gradient of the rock terraces provided some additional soil moisture for vegetal establishment. The rock terraces were constructed in 90 days emp loying the talents of 30 local workers. Rock terraces were built at a rate of 11 m per worker team-day. The standard construction team consisted of a supervisor and 15 construction workers. The cost of rock terrace construction was $48, 000 (US). Although this was a very steep slope and thus the potential for accidents was quite high, precautions such as rope restraints and harnesses and a culture of safety, emphasized daily, precluded any serious incidents.
The rock terraces provided the foundation for costeffectively re-establishing vegetation on this very difficult slope. These efforts encompassed the placement of: (1) 25,000m2 of ENKAMAT™, (2) 45,000m2 of hand seeding, (3) nearly 50,000m2 of top soiling, (4) 32,000m2 of resloping, (5) gully plugs throughout critical areas, nearly 8,000 Polylepis racemosa trees planted and (6) hydro-seeding of 4 ha. This effort has already reduced generated sediment loads and will continue to pay dividends as vegetation becomes more established.
Due to the aggressive slope protection program completed on the fill slope of Canrash Km 94 there is a slight reduction in peak flow and a very large reduction in peak sediment concentration for all design storm events. For example, the predicted peak storm runoff, for the 2- year 24-hour design storm event, is slightly reduced from 0.387 to 0.365 m3/s for the pre-slope stabilization (July 2002) and post-slope stabilization (January 2003) timeframes, respectively. The effectiveness of the slope re-vegetation effort is most strongly reflected in the peak sediment concentration for these two periods. For the 2- year 24-hour design storm the predicted peak sediment concentration is reduced from 350,000 mg/l to 51,000 mg/l for the prior and post slope stabilization timeframes, respectively (Figure 2). This seven-fold decrease in the peak sediment concentration entering Pond 1 is attributed to the initial establishment of rock wall terraces and the effectiveness of vegetation on the fill slope of Canrash Km 94.
The overall effectiveness of the Canrash Km 94 effort is seen, Figure 2, in the predicted peak effluent sediment concentration at the outlet of Pond 4. For the 2- year 24-hour design storm event the peak effluent sediment concentration was reduced from 1,320 to 141 mg/l for the pre- (July 2002) and post-slope stabilization (January 2003) timeframes, respectively. Measured effluent sediment concentration averaged 12 mg/l during the wet season and never exceeded 47 mg/l. Further down-gradient sediment concentration reduction will occur through natural attenuation via an extensive flat and long grass area prior to entering Canrash Lake.
The real benefit of the aggressive rock terrace construction and slope re-vegetation program completed at Canrash Km 94 is seen by the reduced peak sediment concentration and reduced sediment loading predicted for the down-gradient sediment ponds. There is no regulatory peak effluent sediment concentration that is applicable to Km 97 or 94. The necessity of achieving a low sediment effluent concentration is for water quality in Canrash Lake and the down-gradient stream used by the local population. A direct cost saving will be realized by the lower cost of sediment removal in the down-gradient sediment ponds. Long-term slope stabilization, through the use of rock wall terraces and vegetation provides long-term cost reductions and very real long-term water quality enhancements.
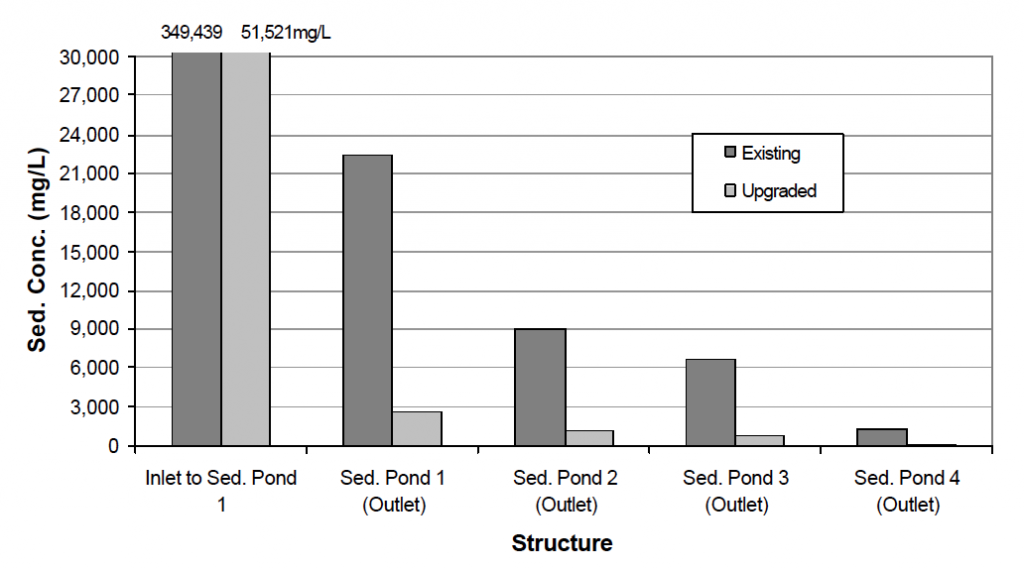
Km 94.
PERFORMANCE OF SEDIMENT PONDS AND INTEGRATING THE NATURAL STREAM BUFFER THROUGH INTERNAL ROCK CHECK DAMS (KM 97 PONDS AND WEEP BERMS)
Alternative designs to improve and performance of the two existing parallel sediment ponds that receive the sediment load generated from cut slopes along approximately 3 Km of access road were investigated. The use of rock structure check dams internally located within the elongated gradient ponds and the addition of a weep berm with rock structures used to distributed runoff to a natural grass stream buffer area proved to greatly enhance system performance with respect to peak flow reduction, overall sediment trap efficiency and effluent sediment concentration.
Figure 3: Plan-view of Km 97 Storm Water Sediment Control System Incorporating Porous Rock Check Dams in Elongated Gradient Ponds and a Weep Berm.
The preferred alternative design:
(1) separates elongated gradient ponds into multichambers through use of internal rock check dams with flow control provided by a pipe, porous rock filled check dams and up-gradient geotextile strips placed on a portion of the rock face,
(2) adds valve-controlled dewatering pipes, near the outlet of the most down-gradient chamber, used to facilitate flow control and nearly complete dewatering enabling higher sediment trapping efficiency and cost-effective sediment removal, and
(3) adds internal flow control rock check dams and multi-outlet (horizontally and vertically located) pipes that discharge to a natural grass filter prior to entering the adjacent stream. The rock check dam configuration enable discharge of runoff to the longest portion of the natural grass buffer thereby enhancing overall system performance.
The six rock check dams placed in the two primary sediment basins, constructed by local workers under the supervision of a local contractor, were approximately 2.75 m in height and filled the width of the elongated ponds (trapezoid, 2m bottom width, 1:1 side slopes, 3m depth). Construction of these 6 rock check dam structures required 18 days and were completed at a cost of $ 4,800 (US). Additionally, three small rock check dams were placed in the channel of the weep berm resulting in 6 construction days and at a cost of $1,200 (US). No lost time accidents occurred during this contractor-directed construction effort. A specialized contractor management safety certification program was implemented in order to improve contractor safety performance.
Passive dewatering of individual pond chambers was provided by a 150mm pipe, that was located nominally 2m from the base space, and via the porosity of the rock check dam through cut openings in the geotextile fabric. Thus the rock check dam was designed such that the size and placement of rock enabled a controlled dewatering rate to enhance sediment pond performance. Future flow control, to accommodate potential clogging by sediment, will be accomplished by removing additional vertical strips of geotextile thus exposing a rock face that is relatively clear of sediment. Hence, traditional rock wall construction techniques were modified by specifying a designed distribution size of rocks that enabled a desired flow control rate. The integration of traditional rock structures, enhanced through design and utilized in nontraditional ways, increased the overall effectiveness of the storm water and sediment control system.
The concept of a weep berm is new to mining operations and was first introduced at a construction site in Georgia that required very low effluent sediment concentration (Warner and Camargo, 2002). The Antamina weep berm was designed to receive valvecontrolled discharge from the elongated up-gradient ponds and passively distribute flow along the length of the weep berm through a series of 25 cm pipes placed at specified horizontal and vertical locations. The weep berm was divided into three chambers using rock check dams constructed by local workers. Flow emanating from the weep berm enters a down-gradient natural grass filter where water is either infiltrated (partially or totally, as a function of discharge rate and spatial location) and/or treated through the filtering action of the vegetation.
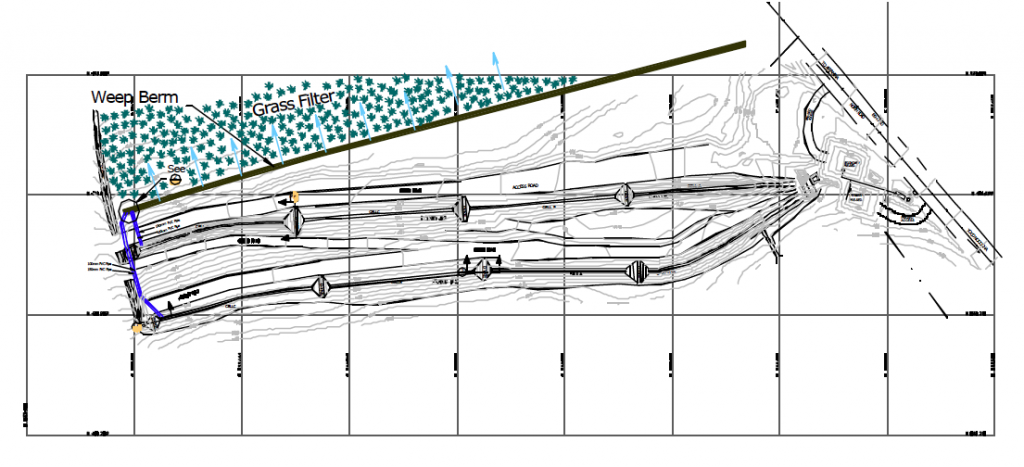
Gradient Ponds and a Weep Berm.
A SEDCAD analysis was conducted to compare the effectiveness of the upgraded design that incorporated rock structures and the weep berm-natural vegetation filter, for various design storms, with the prior dual pond system that existed in July 2002. It should be noted that a comprehensive rainfall analysis was previously competed and 96 % of the historical storm events are less than 20mm for this site. The peak flow for the 20mm design storm is predicted to be reduced from 0.353 m3/s to 0.178 m3/s for duel pond conditions, Figure 4. Whereas, for the system of controls consisting of the duel ponds with rock check dams and weep berm-natural vegetation it is predicted that runoff from the entire storm will be infiltrated within the natural stream buffer. Thus, for 96% of storm events occurring throughout the year the discharge from the weep berm is predicted to be infiltrated within the natural grass riparian zone. It should be noted that this analysis was based on a single design storm and that the overall operation of the control system, based on a temporal series of historical storms, needs to be completed to determine the overall effectiveness of this control system and an optimum operational plan.
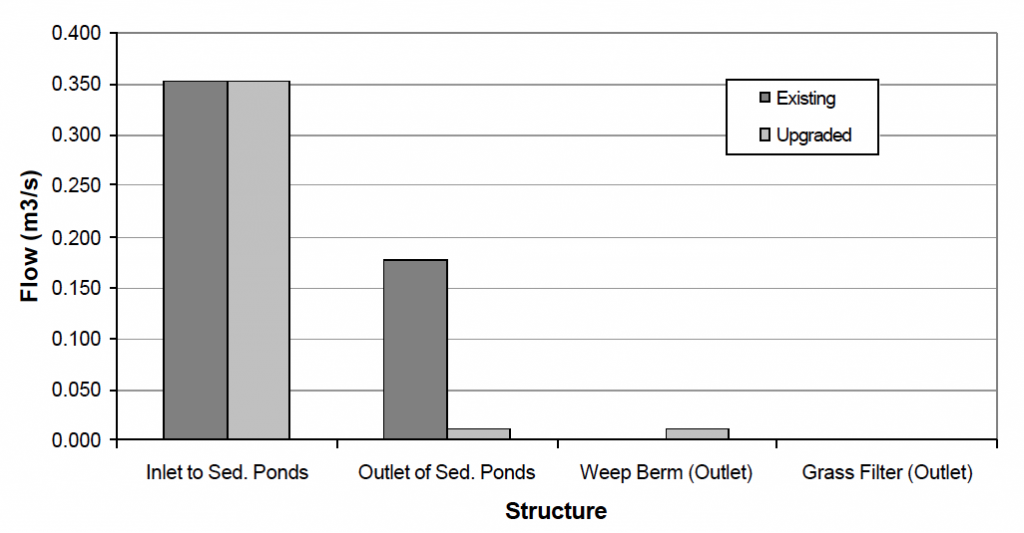
The predicted peak sediment concentration is substantially reduced from approximately 130,000 mg/l to about 300 and near 0 mg/l for the duel pond and upgraded controls, respectively based on the 20 mm design storm, and Sediment Control System for the 20 mm Design Storm (Km 97).
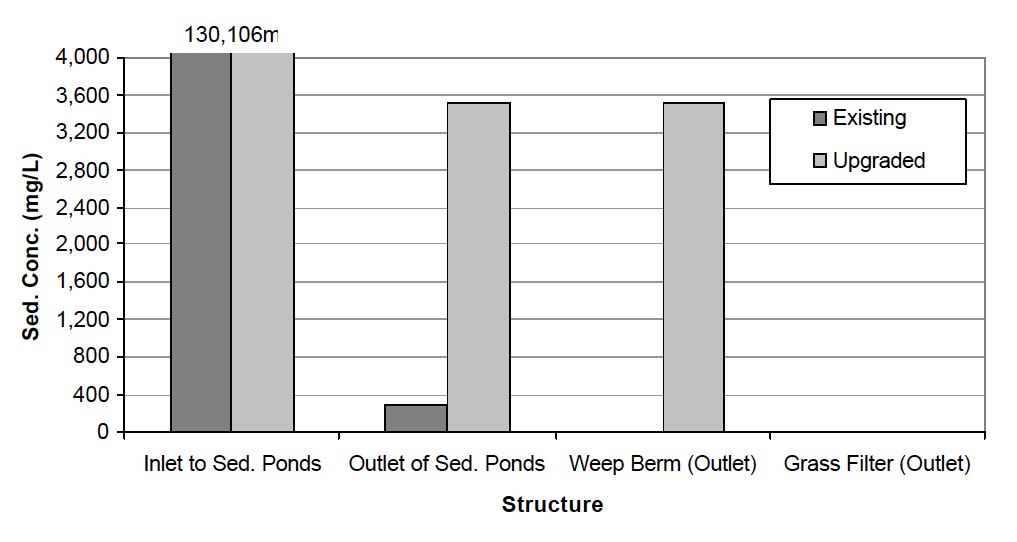
and Sediment Control System for the 20 mm Design Storm (Km 97).
Even the peak flow for the 2-year 24-hour design storm is predicted to be substantially reduced through the upgraded system of controls. For the original duel pond system the peak outflow was reduced by about ½ of the peak inflow, Figure 6. Whereas, under the upgraded system utilizing traditional rock check dam techniques integrated with state-of-the-art storm water control systems the predicted peak flow decreased from 0.933 m3/s to 0.02 m3/s. The implication of this vast reduction in peak flow due to increased infiltration is that the downgradient fluvial system will remain in a stable condition, base flow will be enhanced and effluent sediment concentration will be low.
Monitoring data between January and June 2003 shows that the average daily effluent sediment concentration emanating from the down-gradient natural grass filter is 6.3 mg/l; ranging between 56 and < 1 mg/l. Thus, the addition of rock check dams and utilization of the weep berm provides a greatly enhanced stormwater and sediment control system.
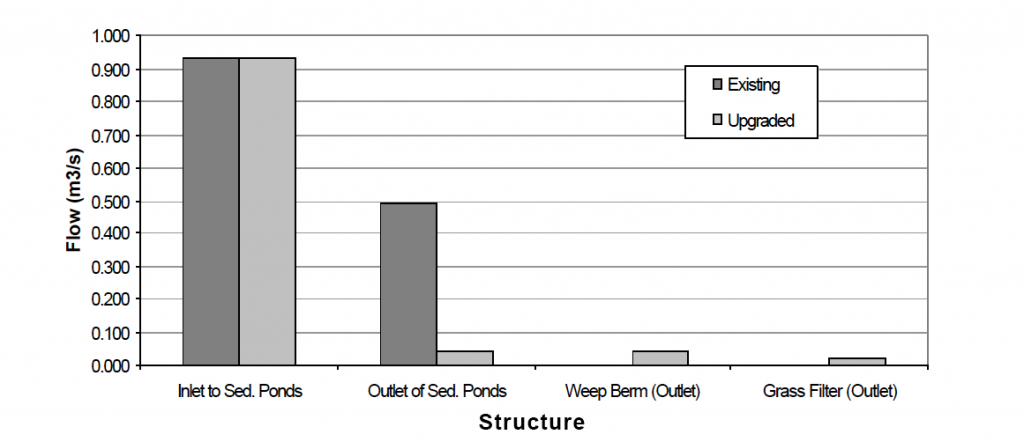
A SERIES OF VARIOUS ROCK STRUCTURES USED AS BEST MANAGEMENT PRACTICES (BMPs)
A 750,000,000 tonne waste dump is planned for the Tucush watershed. A series of three down-gradient sediment basins were constructed prior to site disturbance. Prior to topsoil striping small-scale sediment controls, consisting of rock structures and rock check dams have been built adjacent to topsoil stockpile areas and to control runoff and sediment associated with topsoil strip areas. The spatial extent of topsoil stripping has been minimal focusing on areas that will yield the greatest depth of soil. This method precludes the stripping of extensive areas with very shallow soils located on steep slopes. Steep slopes are protected by natural grass that inherently generates low sediment loads. Thus, three of the major elements of sediment control, i.e. minimizing the area extent to disturbance, protecting steep slopes and integrating the scheduling of stormwater, erosion reduction and sediment controls into the mine plan have been incorporated into the planning and construction of the Tucush waste dump.

Down Gradient Natural Grass Areas.
Several site assessments were conducted at Tucush. Prior and concurrent to topsoil stripping BMPs were established to reduce the generation and transport of sediment to down-gradient sediment basins. Utilization of traditional Peruvian rock structures was emphasized. The specific types of BMPs included rock walls at the base of topsoil stripping areas and immediately down gradient of topsoil stockpiles. Additionally, rock walls, with and without geotextiles, were recommended throughout portions of the stream to attenuated peak flow and slowly release runoff volume to down-gradient natural grass areas in order to infiltrate runoff volume and enhance filtering of sediment-laden flows. All topsoil stockpiles and striped areas were seeded. Typical rock walls, without additional geotextile protection, shown in Figure 7, are used along the topsoil stockpile and to spread flow to natural grassed areas.
The predicted excellent performance of the system of 14 BMPs, for the 20mm design storm, reduced the peak sediment inflow to the up-gradient sediment pond from 240,000 mg/l (without rock structure BMPs) to 15,000 mg/l. The peak effluent sediment concentration, exiting the down-gradient pond, for the 20mm design storm, is predicted to be 50mg/l. Monitoring data substantiates this prediction with the daily maximum and average effluent sediment concentration being 53 and 4.1mg/l, respectively for the timeframe of January through June, 2003.
A total of 13m of rock walls were built within 5 weeks with a crew of 10 at an estimated cost of $ 3,000 (US). The use of relatively simple rock wall technology integrated with the augmented use of geotextiles proved to be a very cost and environmental effective solution that had the additionally benefit of providing substantial employment and took advantage of the knowledge and skills of local workers.
CLEAN WATER AQUEDUCT AND ELONGATED GRADIENT SEDIMENT POND (QUEBRADA ANTAMINA WATERSHED)

Traditional Peruvian Rock Placement Techniques.
The Quebrada Antamina watershed contains the largest potential sources of sediment for the entire project. This is not only the active mining area but contains lake sediments that are being removed, an extensive haul road system, low and medium grade ore stockpiles and auxiliary mining operations. The design of 20 control and conveyance structures was completed for this watershed. Two major permanent structures utilized indigenous rock structure technologies. These include a rectangular aqueduct, 1.5m wide and 1.5m deep, located along the left side of the South Haul Road and a 12-chamber elongated gradient sediment basin (Pond 5) located along the right side of the South Haul Road. Clean water from ninetyplus percent of the undisturbed area of the watershed is conveyed through the aqueduct thereby eliminating a substantial quantity of water that would otherwise require treatment or that would substantial increase the peak flow rate, and hence decrease the effectiveness of the control system. The clean water aqueduct, pictured in Figure 8, was designed to convey the 100-year 24-hour design storm.
Pond 5 consists of 12 chambers divided by rock check dams with the up-gradient face covered by a geotextile. Flocculent is introduced prior to the most upgradient chamber and is rapidly mixed to achieve flocculent-to-particle contact. The first chamber is 100m in length and is designed to increase the size of flocculated particles. The remaining cells provide backwater for flocculated-particle settling. Stormwater detention is most evident for the smaller storm events. Discharge between up- and down-gradient cells is controlled by 5 pipes that are placed within the rock check dams at three different elevations. Additional flow control is provided by removing sections of the geotextile.
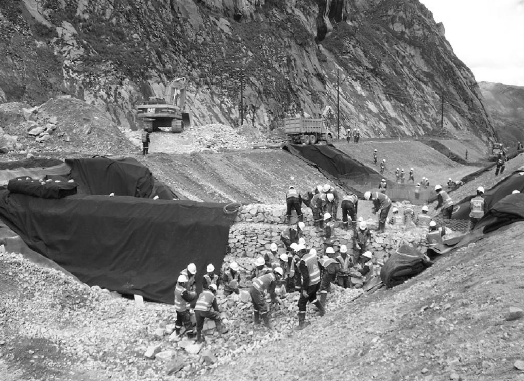
Large Rock Check Dams Segmenting Pond 5 in Quebrada
Antamina to Enhance the Flocculation Treatment System.
The design of Pond 5 provides many advantages compared to traditional sediment basin designs. The controlled-flow passive dewatering systems facilitated by the rock check dams provide a volume for complete or partial containment of the next storm event. Besides enhancing the effectiveness of the flocculation system the design of Pond 5 will decrease the peak flow, increase sediment trap efficiency and decrease effluent sediment concentration. Perhaps as important, the design of Pond 5, with its elongated narrow structure, enables highly effective sediment removal by construction equipment that can be operated along berms located on both sides of the elongated sediment pond and readily transfer excavated, and relatively dry, sediment to trucks located along the haul road. It is predicted that Pond 5 will provide excellent effluent quality for the design storm. A portion of Pond 5, illustrating the rock check dams that segment the pond and provide flow control along the sediment basin is shown in Figure 9.
Construction of the 12 massive check dams (bottom width 20m and depth 3m) was completed by 120 local workers in approximately 5 weeks. Again, due to a diligent safety program that was embraced by workers, no lost time accidents occurred.
LESSONS LEARNED
The Antamina experience shows that incorporating traditional technologies and knowledge into storm water, erosion reduction and sediment control systems pays dividends. Not only is a cost- and environmentally effective system achieved but also a practical partnership is realized that promotes involvement of stakeholders and effectively increases environmental awareness of communities in the area of influence.
Seeking out opportunities to employ local workers and incorporating their ancestral knowledge about constructing rock structures as terraces and for the conveyance of water results in monetary gain for workers. Increasing worker’s knowledge about safety provides a triple dividend; a safe mining operation is achieved, worker’s incorporate some of these safety procedure in their everyday life and worker’s share this knowledge with family and neighbors. As local workers build rock walls and channels they gain a realization of Antamina’s commitment to protecting the environment. This is no longer just a vague notion, a real tangible example that mine runoff that has been cleaned through their efforts; is the same water that they use for irrigation and domestic water supply. They share this first-hand knowledge about the mine’s concern for erosion, reclamation and environmental protection with community neighbors.
Pride in a job well done that helps to improve the community’s water is seen and heard from local workers. Very often their comments and recommendations improve the overall performance of erosion and sediment controls.
Employment of local workers is one way to implement the concept of a “triple bottom line” where – economics, environment and social responsibility are key components of business. Antamina’s experience in aggressively seeking opportunities to employ local workers and draw upon their knowledge has proven to be a positive experience. Antamina continues to employ local workers in implementing and maintaining storm water, erosion reduction and sediment control systems.
REFERENCES
Manual
Warner, R.C., P.J. Schwab and D.J. Marshall, 1998, SEDCAD 4 for Windows 95/98 & NT: Design Manual and User’s Guide. Civil Software Design, Ames, IA. 125 pages plus CD.
Reports
1. Warner, R.C. and Francis Collins-Camargo, 2001, “Erosion Prevention and Sediment Control Computer Modeling Project”, Chattahoochee-Flint Regional Development Center. Franklin, GA.
2. Warner, R.C. and Sebastian Torrealba, 2003, “Design and Evaluation of Storm Water, Erosion and Sediment Control Systems for the Antamina Mining Complex”, Technical Report for Compañia Minera Antamina.
Journal article
Botts, S.D. The Antamina Project: The Challenge of Sustainable Development in Peru. 2003 SME Annual Meeting. Mining and Sustainable Development Case Studies. 25 Feb 2003.
This article can be downloaded in its entirety in PDF format.